Ph.D. Research:
In my dissertation research, I investigated the drivers of growth in white spruce (Picea glauca) growing at Arctic treeline in the Brooks Range, AK, USA. Each chapter is linked by a common pair of questions: How do temperature and water availability influence growth of white spruce at Arctic treeline; and how do these relationships change between saplings and trees?
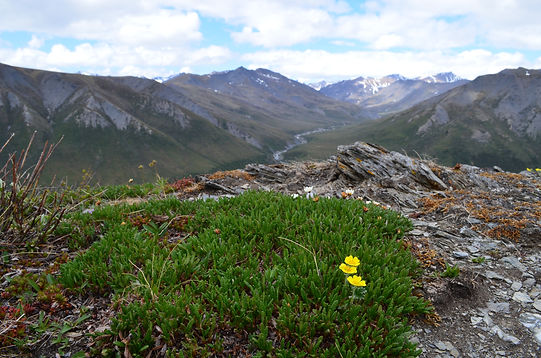
What is the Arctic treeline?
The Arctic treeline represents the transition from the coniferous boreal forest to the south and treeless Arctic tundra to the north. Arctic treeline spans the circumpolar region crossing 13,400 km through the United States (Alaska), Canada, Iceland, Norway, Finland, and Russia. When observed from air or space, Arctic treeline may look like a continuous line. However, on the ground, this interface between ecosystems is complex and varies in space and time due to numerous local and regional conditions.
Chapter 1:
Growth increases but regeneration declines in response to warming and drying at Arctic treeline in white spruce (Picea glauca)

In Chapter 1, I used historical records of temperature and precipitation, present-day age structure, and tree ring records to investigate the relationships between climate change, treeline behavior, and tree growth during the 20th century. I found that a warmer but significantly drier climate beginning circa 1975 was strongly associated with decreased regeneration of saplings, suggesting treeline remained stationary despite climate warming. This finding supports our hypothesis that once temperature limitation is alleviated, water availability acts as a secondary control on growth at Arctic treeline in saplings. However, our data also showed a significant increase in growth of mature trees after 1975. These opposite responses of saplings and mature trees support our hypothesis that the secondary control of water availability is more severe for saplings than mature trees. Further, the tree ring records revealed temperature divergence after circa 1990, suggesting additional drying in the future will begin to negatively impact growth of mature trees. We recommend considering life stage and the interactions between temperature and moisture availability when making predictions about growth at treeline in a changing climate.
This chapter is in peer-review at the NASA ABoVE special issue of Environmental Research Letters and is available as a pre-print here.
Figure caption:
(Top) The climate became significantly warmer at Arctic treeline in central Brooks Range as shown by the climate index developed by Barber et al (2000). The index differentiates between relatively cool, wet years (positive values) and warm, dry years (negative values). A loess smoothing curve (blue line) with 95% confidence intervals (grey shading) shows the overall trend through time.
(Middle) Tree ring width chronologies with two types of detrending (modified negative exponential and two-thirds spline) of white spruce trees at Arctic treeline in the central Brooks Range, AK show growth increased c. 1975 by an average of 52%. Y-axis shows the ring-width index (RWI), or standardized ring width after detrending.
(Bottom) Age structure of white spruce trees in the study area in 2011 show regeneration of saplings decreased c. 1975. Columns represent the mean number of individuals in each age class, where error bars represent the 95% confidence interval. Note, that trees below 0.3 meters tall were not detectable by aerial lidar which limited the age structure to those established before 1995.
The vertical red line indicates the year 1975, when shifts occur in climate, individual, and population growth.

Chapter 2:
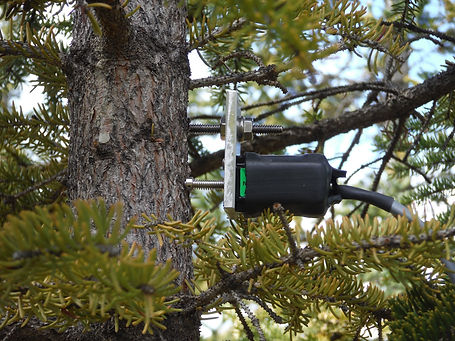
In Chapter 2, I explored the present-day relationships between tree life stage, temperature, moisture availability, and radial stem growth by examining the response of intra-annual radial stem growth rate to changing environmental conditions at Arctic treeline. To do so, I used a generalized linear mixed effects model to predict radial stem growth rate using air temperature, vapor pressure deficit (VPD), volumetric water content, and their interactions as drivers. Diameter at breast height (DBH) is added to the model as a proxy for tree life stage. Our results sugest that VPD and VWC are influence growth rate more than air temperature. In addition, while low VPD increased growth in both saplings and mature trees, the effect was greater in mature trees. These results support our hypothesis that trees at our Arctic treeline site are increasingly water-stressed with rapid warming, and that tree size plays an important role in determining the growth response. The result of this study suggests that we may be currently witnessing that the transition towards water-limitation predicted from Chapter 1.
Temperature vs moisture: dissecting the relative influence of temperature, vapor pressure deficit, and soil moisture on intra-annual radial stem growth at Arctic treeline
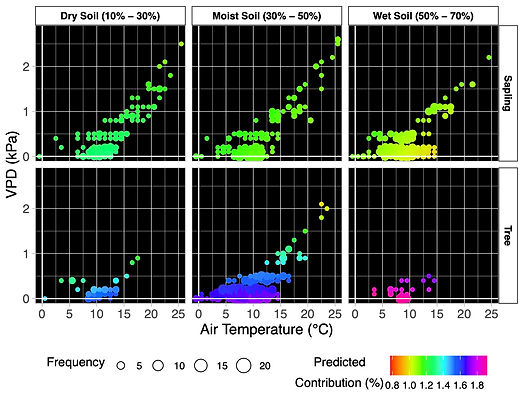
Figure Caption. Heat map of the tree growth model showing the relationships between white spruce growth rate and the weather variables at Arctic treeline, AK. Interactions between predicted growth rate (color), temperature (x-axis; °C), relative humidity (y-axis; %), and three levels of soil moisture (columns; % VWC) impact white spruce saplings (top row; DBH = 6.6 cm) and trees (bottom row; DBH = 18.4 cm) differently. Relationships are shown for growth on June 10 (DOY 162) but were similar throughout the growing season. Low, moderate, and high values for soil moisture were chosen to represent the range of conditions experienced during the growing season. The size of the points describes the frequency (number of 20-min timestamps) of that combination of weather conditions that occurred during the growing seasons of 2018 and 2019.
Chapter 3:
Extreme cold and subsequent thermal accumulation provide the environmental cues for initiating radial stem growth at the Arctic treeline: A dendrometer study of white spruce (Picea glauca) in central Alaska, USA
In Chapter 3, I explored how the onset of intra-annual radial stem growth will change in response to climate change. Using a Bayesian analysis, I compared the process triggering the onset of radial stem growth and the type of trigger (i.e., air temperature, soil temperature, or both). In this chapter, I used soil temperature as a proxy for water availability in spring, as discontinuous permafrost will not allow liquid water to be available for plant uptake until soil temperatures exceed freezing. Our results suggest that air temperature was a better predictor of the onset of radial growth in spring than either soil temperature or a combination of both. I also found that a combination of extreme cold followed by heat accumulation triggers radial growth. These results suggest that air temperature is more important than water availability in dictating the start of the growing season.
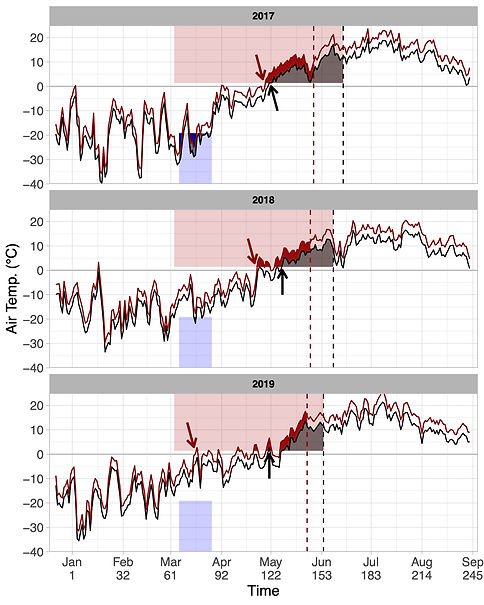
Figure Caption.
Graphical representation of the difference in onset of radial stem growth in a white spruce tree after the addition of 4°C (dark red line) to the actual daily mean temperature (black line) in 2017 (top), 2018 (middle), and 2019 (bottom). The onset of radial stem growth is represented by vertical dashed lines. The heat accumulation period is represented by the red-shaded region and the chilling period by the blue-shaded region. Red-shaded areas under the temperature lines represent the accumulated temperature above the threshold, until the onset of radial stem growth is triggered. Heat accumulation under 4°C warmer climate begins earlier (red arrows) than under current conditions (black arrows). Meanwhile, the number of days below the chilling threshold, during the chilling period (blue shaded areas above the temperature lines, top row) decreases with 4°C warmer climate.